Your browser is not supported.
For the best experience, please access this site using the latest version of the following browsers:
By closing this window you acknowledge that your experience on this website may be degraded.
Radar Corner
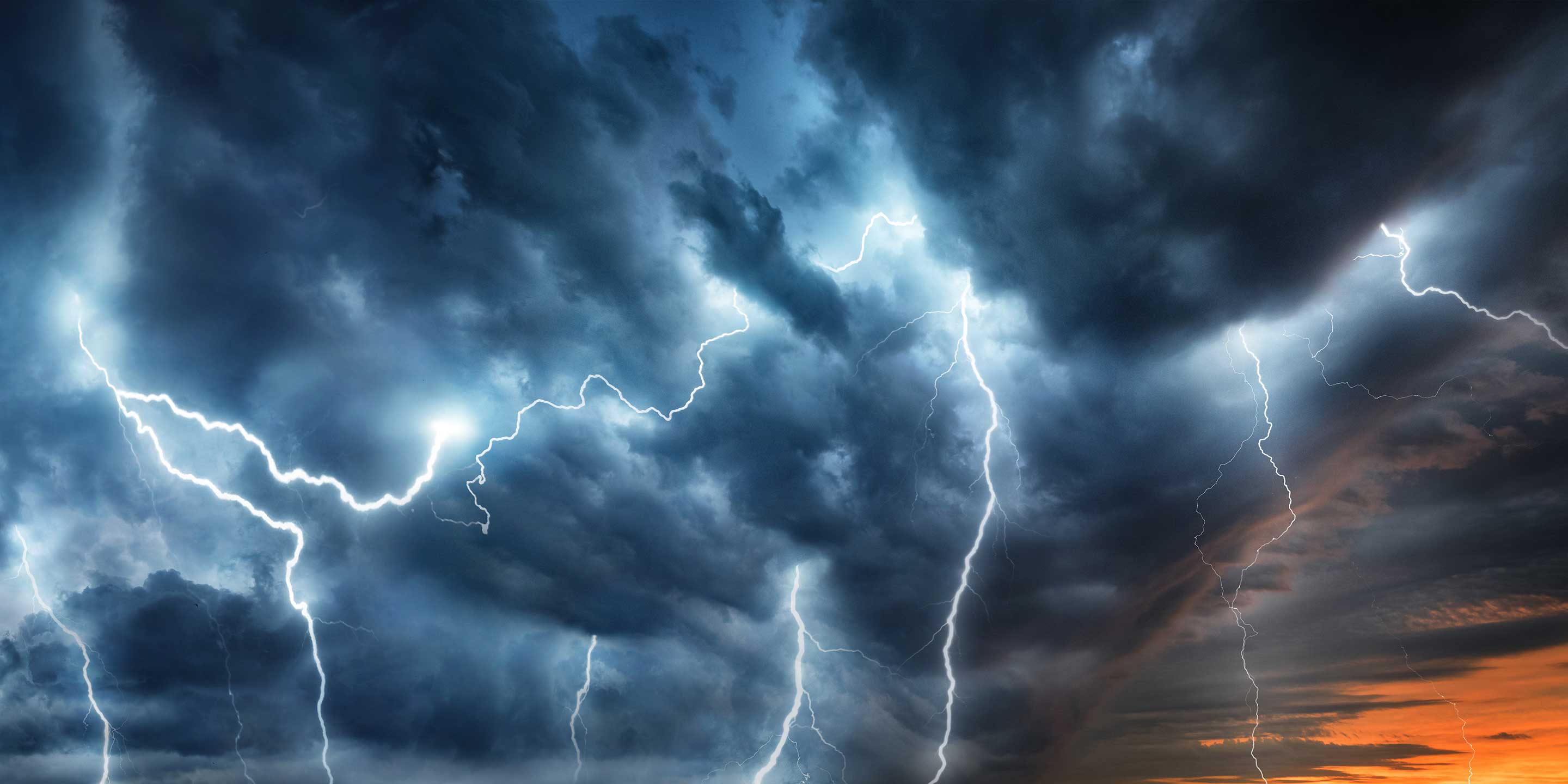
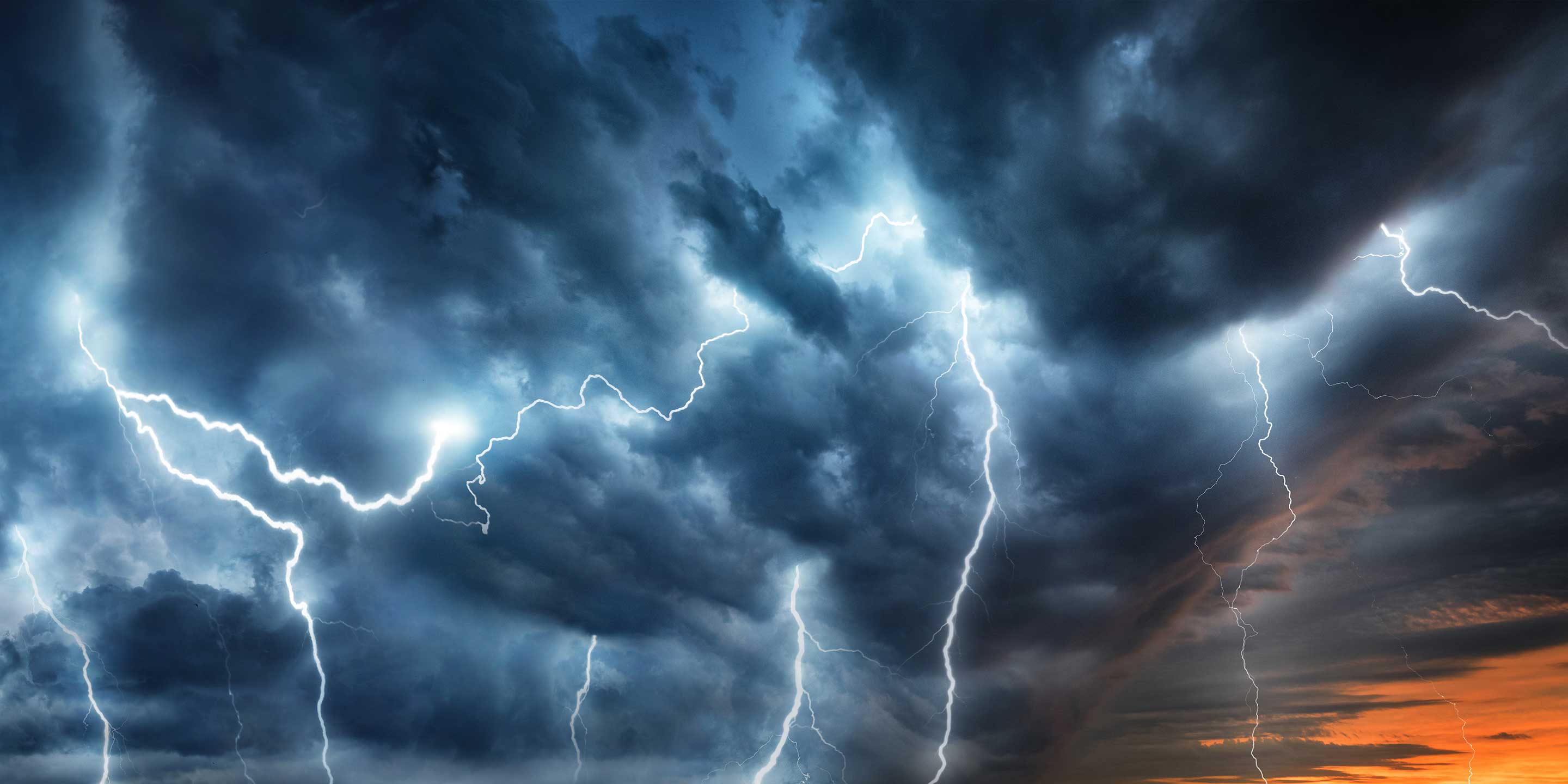
Radar Corner
Follow-Up to Understanding Windshear Detection Systems
In our recent “Understanding Windshear Detection Systems” series, some readers astutely noted that we did not define what a windshear “event” is. We described the microburst phenomenon and used words like “when the event was detected.”
One of the breakthrough moments in the development of forward-looking windshear detection systems was the simple-yet-eloquent definition of F-Factor or Hazard Factor. The Air Mass F-Factor mathematically describes the effect of the local wind velocities and can be estimated by the radar, while the aircraft F-Factor mathematically describes the excess thrust. Put simply, if an aircraft has an F-Factor of 0.15 and the windshear has an F-Factor greater than 0.15, it’s a bad day. For example, if an aircraft was traveling at 150 knots and encountered a windshear with an F-Factor of 0.15 for 24 seconds (1 NM), it would lose 911 feet of altitude if an escape maneuver was not initiated.
The Air Mass F-Factor can be estimated by the radar:
Where:
△Wx = radar estimated change in horizontal wind velocity
△x = distance between horizontal wind velocity estimates
g = acceleration due to gravity
Vg = ground speed (assumed constant 150 knots)
Va = true airspeed (assumed constant 150 knots)
β(z) = a scalar function of resolution cell altitude AGL
Those of you still awake at this point can see two things: First, why we avoided describing this in the initial article; and second, that the Air Mass F-Factor consists of two terms. One is the horizontal wind velocity, and the other is an inferred vertical wind velocity. Doppler radar can only measure the speed of the raindrops in the horizontal plane, or the raindrops moving toward and away from the aircraft when the rain hits the ground and mushrooms out radially. The radar cannot measure the speed of the raindrops in the center vertical downdraft. NASA conducted studies and provided manufacturers with an empirical model β(z) that infers vertical wind velocities based on the measured horizontal velocities.
Figure 1. Microburst |
During development, different types of alerts, displays, and maneuvers were considered, including a display of the actual wind velocities. One of the most important concepts is that it is the advanced warning time that saves the aircraft! This time is used to increase power and clean the aircraft up, increasing the aircraft’s energy state and climbing so that you encounter the windshear at a higher, more-survivable altitude and where the windshear has not mushroomed out radially. For this only a simple visual and aural alert are required. NASA and the FAA evaluated several different maneuvers for windshear recovery as well. In pilot trials, the difference between these maneuvers varied by only 20 feet. But when these maneuvers were delayed by just 5 seconds the average altitude loss increased by 300 feet! It is the advanced warning time that saves the aircraft.
Let’s put our new knowledge about F-Factors into a real-world example, shown in Figure 2. A three-engine air transport aircraft is on a normal final approach passing through 1,200 feet AGL traveling at 140 knots. As it does, the air crew receives an audible alert from the Honeywell Forward-Looking Windshear Radar, "Windshear Ahead, Windshear Ahead." They also receive visual alerts. They immediately add power and climb at a constant air speed and a constant climb rate of 1,000 feet/minute to 2,050 feet AGL before encountering the microburst at point A. At that location in the microburst, the air mass F-factor is 0.286. During this time the crew has had time to prepare the aircraft for the encounter, they have adjusted the aircraft flaps for go around, retracted the landing gear, and added power. This action gives them an aircraft F-factor of about 0.104.
As they pass through the microburst, they have an overall System F-factor of -0.182, which translates to a descent flight angle of -10.5 degrees. At 140 knots and that flight path angle, they lose 500 feet and emerge from the back side of the microburst at point B, at 1,550 feet AGL. They then finish executing a safe go around.
Figure 2. Real-World F-Factor Example |
Now let's see what could have happened if the crew had not been made aware of the microburst. They would have entered it at point C (shown in Figure 2). The F-factor of the airplane configured for landing is typically 0.042. The average F-factor of the air mass at that location is 0.282. After subtracting the F-factor of the air mass from the F-factor of the aircraft, a net System F-factor of ‑0.240 remains. That translates to a descent angle for the aircraft of -14 degrees. Since they began this second scenario at only 600 feet AGL, the aircraft would contact the ground approximately 2,400 feet farther forward in its flight path about 11 seconds later.
Over the years in training classes pilots have commented to me that they executed a go-around and it didn’t seem that bad. They thought they might have been able to land. And you might be tempted to underestimate the danger of the microburst having successfully negotiated it. You should not be deceived by what appears to be a minor encounter and end up thinking that you could have landed the airplane despite the presence of the microburst. Remember that it was the increased energy-state of the aircraft and the high altitude point of entry that allowed you to easily fly through it. Had it not been for those conditions, you would not have been able to successfully negotiate it. Remember that the effects of a microburst are much more dangerous when the point of entry is near the ground. At low altitude, you are faced with three difficult conditions:
1. The aircraft is configured for high drag characteristics that reduce its climb performance.
2. The air mass of the microburst has mushroomed making its danger zone considerably wider.
3. You have less altitude to perform an escape maneuver.
Rather than having individual options for each type of aircraft, forward-looking windshear detection systems use some basic assumptions for approach speeds and even aircraft hazard factors (F-Factors). Currently, the same assumptions are used for air transport and business jet aircraft. We use 150 knots for the determination of hazard factors for all aircraft, even smaller business aircraft. NASA would need time to determine if there is a “better” speed (or “better” thresholds) to use for smaller aircraft, but that is not currently on the docket.
As you leave transport category aircraft and move into lighter aircraft, you get:
– Higher thrust-to-weight ratios
– More maneuverability
– Easier escape, even if its speed is slower
So, the transport thresholds (0.085 and 0.13) are more conservative for these smaller aircraft, but that should be okay.
F-factor:
v = Aircraft true airspeed in meters per second (m/s) = fixed at 150 knots (77.2 m/s) for calculation of F-factor. (Although this is more representative of a heavyweight aircraft, this value can be used for all aircraft types. In general, it will tend to yield slightly higher, and thus more conservative, F-factor values than would result from lower approach speeds.)
One last word of caution is necessary before we leave this subject. If you find yourself in a situation where you are going to fly through any part of a microburst, even at a higher altitude, prepare yourself for significant roll effects. The wind velocity gradients are very high in and around a microburst and, therefore, develop high roll force imbalances across the wing surfaces.
Program Pilot Stephen Hammack supports Honeywell Apex and radar for Flight Technical Services. He can be reached via email at Stephen.Hammack@Honeywell.com.