Your browser is not supported.
For the best experience, please access this site using the latest version of the following browsers:
By closing this window you acknowledge that your experience on this website may be degraded.
Radar Corner
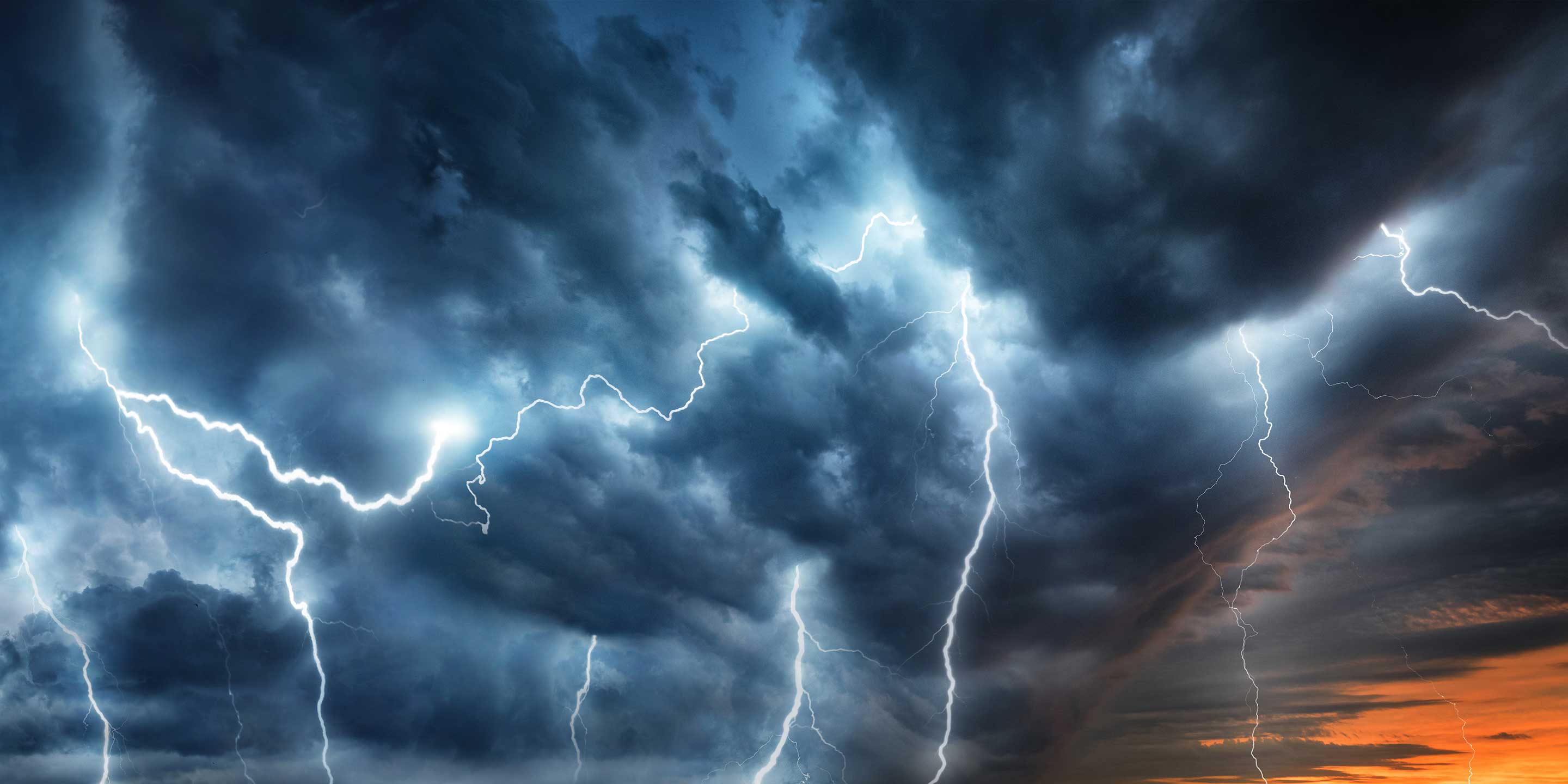
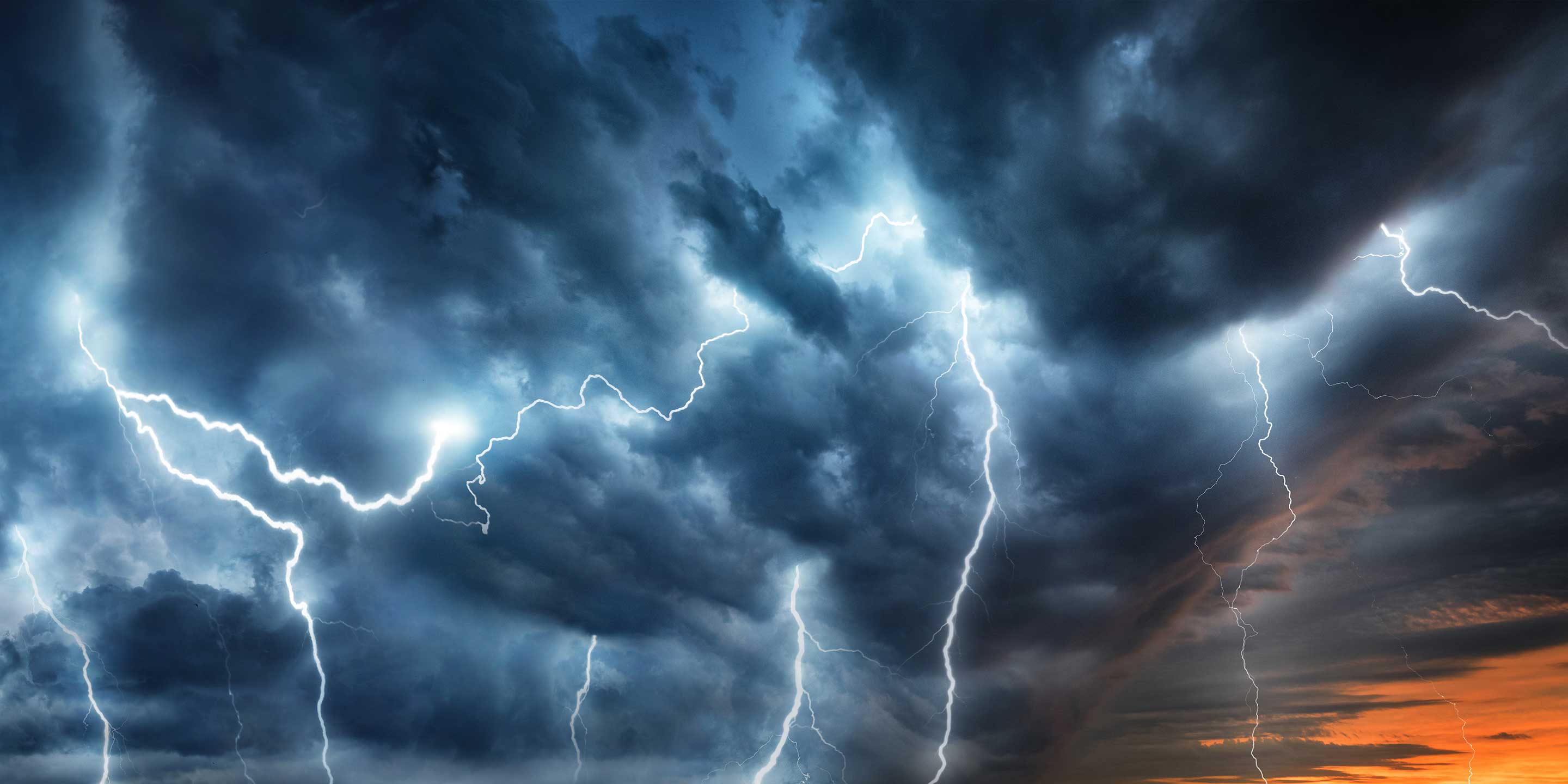
Radar Corner
Understanding Airborne Windshear Detection Systems, Part Three
The last two articles in this series discussed the discovery of the windshear phenomenon and the development of windshear detection systems. The series will conclude by fast forwarding a few years (1996-1997) from the last article to look at an interesting engineering problem.
Overall system performance of the RDR-4B Forward Looking Windshear Detection System (FLWS) had exceeded all certification requirements. One of the key performance metrics is the combined nuisance and false alert rates. In the present context, all unjustified alerts, regardless of reason or causality, are classified as nuisance alerts. On average, the nuisance alert rate for the RDR-4B system has been consistently close to one alert per one hundred thousand takeoffs and landings, or ten times better than required. This low rate has been a significant factor in overall flight crew acceptance and confidence in the system. There was, however, a localized problem at Kansai International Airport in Osaka, Japan, where the nuisance alert rate was significantly higher than average.
As reported by the flight crews who regularly service Kansai, there were two unusual characteristics about the nuisance alerts. First, they were strongly associated with particular runways and flight phases – takeoffs on Runway 06 and landings on Runway 24, though no alerts had been reported while landing on Runway 06 or taking off on Runway 24. The second unusual characteristic was a correlation with wind conditions; alerts were less likely with strong winds. Theories abounded, including everything from large exhaust fans to satellite dishes. Ultimately the Convair-580 was flown to Osaka for data collection and analysis. It took many flights to collect data for takeoff and landing on different runways and under different wind conditions.
The most important factor at Kansai was determined to be the Sky Gate Bridge connecting the airport, built on an artificial island in Osaka bay, to the mainland. The Sky Gate Bridge is a very large structure – a double-decker bridge supporting a six-lane highway on the upper deck and a two-way railway track on the lower deck. Throughout most of its span, the two decks are inter-connected with massive steel girders, some of which are visible in the photograph taken from the Kansai terminal building. When viewed by radar at low grazing angles the bridge appears to be comparable in size to several super-tankers placed end-to- end, exhibiting a normalized radar cross section 100 times more reflective than average normal urban environments like LaGuardia and Boston Logan. The Sky Gate Bridge dominates the Kansai radar environment.
To understand how the Sky Gate Bridge was causing nuisance messages, one must first understand the radar beam, sidelobes, and a little about Doppler. Remember that engineers try to place all the radar’s energy in the main beam, but there are always sidelobes. Sidelobes are unavoidable and is just energy going somewhere other than the main beam. At high altitude they are not usually an issue, but at low altitude (e.g. during takeoff and landing) they can bounce energy off of the ground or nearby structures.
The other element is how Doppler processing works. Objects directly in front of the radar show as their true speed (cosine of 0° = 1). At 90° the speed is zero (cosine of 90° = 0) and it varies in between.
The image below converts speed into shades of green. Darker green is near 0 knots and faster speeds are shown as lighter colors. Items picked up in the main beam are shown at their true speed. Items picked up in the sidelobes vary with the cosine of the angle.
Here is what a windshear looks like in the Doppler domain when it is picked up in the main beam and the sidelobes. The raindrops coming towards the aircraft look faster (light colors) and those moving away from the aircraft look slower (darker colors). Of course, the system must remove any aircraft movement (groundspeed) from the data.
The picture below shows Kansai airport and the orientation of the Sky Gate Bridge.
Finally, here is a look at what happens when the sidelobes hit the Sky Gate Bridge. There are several things at play here. The large metal bridge dominates the radar environment at Kansai. The orientation of the bridge and the runways causes returns to be picked up in the sidelobes. Even though the bridge is stationary, there appears to be movement because it is picked up in the sidelobes. And finally, the bridge is the same length as a typical windshear.
And the big finale… if the two Doppler signatures are placed side by side, it is apparent that the signature of a typical microburst is almost identical to the signature from the Skygate bridge. Fortunately, now that the problem is known, it can be addressed. With the RDR-4B, engineers had several options. They ended up using what they called an “upper bar scan” to validate that there was a rain shaft above the outflow causing the microburst. Because the airport is on an island, it won’t ever have a dry microburst scenario, so engineers could also look at the location and adjust the system sensitivity.
One might ask what the wind has to do with all of this. It turns out that on calm wind days, the radar energy bounced off the smooth water, hit the bridge, and was reflected back to the radar which caused the nuisance alert. On days when the wind was stronger, it caused waves in Osaka bay. The waves scattered the radar’s energy, which meant much less of it reflected off the bridge.
Fast forward a few more years to Sydney Kingsford Smith airport in Australia. A major airline based in Singapore was getting frequent nuisance windshear system failures while performing the windshear system test before departure. Their gate is tucked into a corner and speculation is that the failures are caused by radar reflections from the corner walls, interference from cell phones, hand held radios, etc.
Honeywell offered to take test equipment to the airport and determine the root cause of the interference, but was turned down multiple times because security wouldn’t allow it. This went on for quite a while and caused considerable pain for the airline. Flying between Australia and Singapore means crossing the Inter-tropical Convergence Zone (ITCZ) and the South Pacific Convergence Zone (SPCZ), both which are very active weather producers. So, a failed radar test is a no-go item and the airline was required to stock additional spares and delay flights while the units were replaced. This became unacceptable and the airport authority “hired” a firm to come in and determine the source of the interference.
After multiple days of testing and analysis the source was determined. On the other side of one of the walls was a men’s bathroom. The toilets, like most, had motion detectors that sense motion and flush the toilet automatically. These sensors used radio frequency to detect motion, but unfortunately they created harmonic frequencies in the same frequency band used for windshear detection. So if by chance the windshear test was being run at the same time the sensor was transmitting, pilots would get a windshear fault.
Honeywell’s new RDR-4000 IntuVue weather radar is becoming available on more and more business and commuter aircraft. In addition to providing windshear detection for this market, it introduces many other significant advancements in radar technology including automatic modes, vertical profile of weather and terrain, enhanced long-range turbulence detection, hail and lightning prediction, analysis modes, ground clutter elimination, and much more.
Previously only available on military radars costing millions of dollars, the RDR-4000 also includes Pulse Compression. Pulse Compression provides both longer range and higher resolution that were exclusive on legacy radars. In addition, there are different types of Pulse Compression used. One type reduces azimuth sidelobes, making the RDR-4000 less susceptible to nuisance alerts. The picture below shows Honeywell’s RDR-4000-equipped B757 doing data collection at Kansai International Airport.
This concludes Direct-TO's three part series on understanding airborne windshear detection systems. The entire series is now available on the Honeywell Pilot Gateway, and will be stored in the Direct-TO Newsletter archive.
Program Pilot Stephen Hammack supports Honeywell Apex and radar for Flight Technical Services. He can be reached at Stephen.Hammack@Honeywell.com.