Your browser is not supported.
For the best experience, please access this site using the latest version of the following browsers:
By closing this window you acknowledge that your experience on this website may be degraded.
Radar Corner: Airborne Windshear Detection, Part One
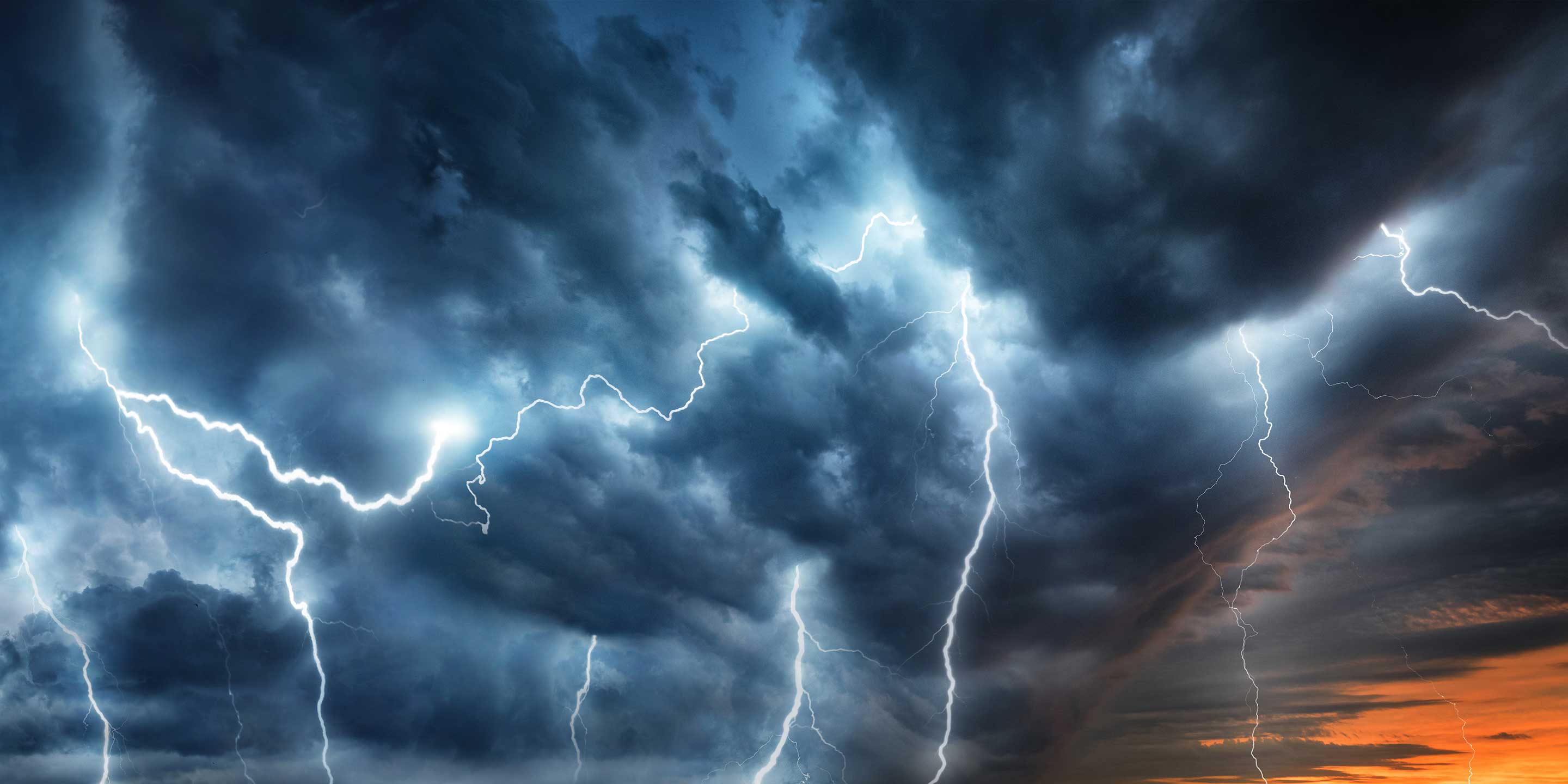
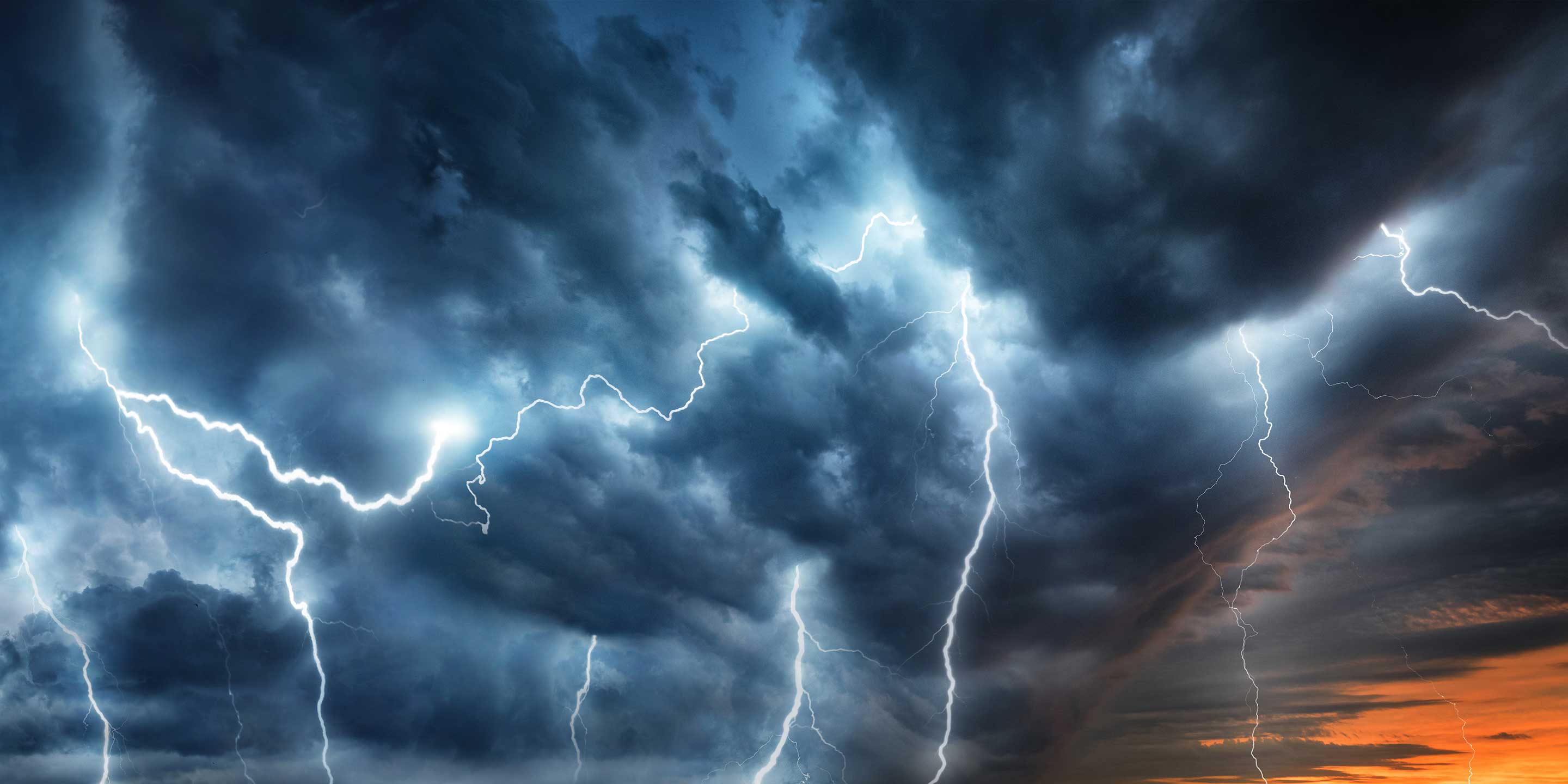
Radar Corner
Understanding Airborne Windshear Detection Systems, Part One
Thanks to the capabilities of Honeywell’s RDR-4000 IntuVue Weather Radar System, more and more aircraft are being equipped with Forward Looking Windshear Detection capability. This article will discuss the discovery of the windshear phenomenon, after a few terms are introduced.
While most aircraft have reactive windshear systems installed, this article focuses on Predictive Windshear Systems (PWS), more aptly named Forward Looking Windshear Detection Systems (FLWS). The term “Forward Looking” is preferred over “Predictive” because there is nothing predictive about them. These systems use the on-board weather radar to look ahead of the aircraft and measure atmospheric conditions to provide alerts to the crew. Although these systems are new to business and commuter aircraft, they have been certified on commercial airliners for quite some time. The first FLWS system was certified by Honeywell on a Continental Airlines B737 in 1994.
The Microburst
There are many types of windshear, but this article concentrates on a specific type called a microburst.
In 1974, severe weather spawned a super outbreak of 148 tornadoes in Beckly, West Virginia. Dr. Theodore Fujita of the University of Chicago was conducting an aerial survey of the aftermath when he noticed some peculiar damage patterns in the fields. Unlike the circular or swirling patterns normally associated with tornadic activity, he observed starburst patterns. It looked almost as if someone had placed an invisible bomb in the center of the field, with the damage pattern occurring radially outward from the center.
Dr. Fujita hypothesized a microburst has a vertical cylindrical shaft of rain cooled air. This cooler, denser air is heavier than the underlying warmer air. This denser state causes the shaft of air to plunge from storm top altitudes downward towards the earth at significant velocities. Upon encountering the ground, the air mass mushrooms radially outward in a horizontal direction.
Microburst Reaching the Ground and Moving Radially Outward
An aircraft flying through a microburst on approach to landing would first encounter increasing headwinds, which creates additional lift causing the aircraft to go above the glideslope. The pilot’s natural tendency would be to reduce pitch attitude and power to re-establish the glideslope. As the aircraft encounters the downdraft in the center of the microburst, the descent rate increases dramatically. As the aircraft passes through the downdraft to the tailwind portion, it experiences a serious loss of lift due to the decreased airspeed. All of this occurs when the aircraft is close to the ground, flying with reduced power, and configured for landing with gear and flaps down.
Final Approach Segment Flown Through a Microburst
Unfortunately, Dr. Fujita’s theory was welcomed as warmly as Christopher Columbus saying the world wasn’t flat. It wasn’t until August 2, 1985, when Delta Flight 191 crashed in Dallas-Ft. Worth that Dr. Fujita was proven correct. The NEXRAD Doppler radar site at DFW provided the evidence that microbursts did exist.
Once the threat was identified, the FAA, NASA, National Center for Atmospheric Research (NCAR), and the industry responded quickly to provide tools and training for pilots. Visual cues for identifying microbursts were provided and the windshear escape and recovery maneuver was developed. Industry also developed and deployed ground-based Low Level Windshear Alerting Systems (LLWAS) which utilizes wind sensors placed around the field to provide alerts to the tower. But more still needed to be done and several studies were conducted to learn additional information about microbursts.
Two major studies, the NIMROD (Northern Illinois Meteorological Research on Downburst) and JAWS (Joint Airport Weather Studies Project) provided significant information to the aviation community. In the NIMROD study 50 microbursts were observed in just 42 days, and in the JAWS study 186 microbursts were observed in just 86 days. Based on this work, downbursts were divided into macrobursts and microbursts according to the extent of their horizontal windfields.
Microbursts are 2.5 miles wide or less which is on the same order as a typical runway and are more of a concern to the aviation community due to the tighter wind field gradient over that runway length. It was also found that microbursts are not always associated with severe weather and there may be little to no rain evident at the surface (dry microburst).
It was also found that the average duration for microbursts in these two studies was between 2.8 and 3.4 minutes. So, it is easy to see why one aircraft can have a relatively benign approach and landing while another just a few minutes later can encounter entirely different conditions. It also shows how quickly warnings from ground-based windshear warning equipment (LLWAS) need to be relayed to airborne flight crews, and, why there was a need for an airborne sensor.
Wet Microburst
Dry Microburst
At the time the only viable technology available for airborne sensors were reactive windshear systems. Reactive systems use aircraft inputs (airspeed, groundspeed, altitude, accelerometers, AOA, etc.) to detect a degradation in performance. Reactive systems were a significant advancement but the aircraft must already be in the microburst and experiencing a degradation in performance before pilots receive an alert. Depending on the altitude at which windshear was encountered, recovery may not be possible. NASA studies had shown that as few as 10 seconds of warning prior to encountering a microburst significantly improved chances of recovery.
With the 1990s came the advent of Digital Signal Processors (DSPs) and the needed processing power for forward-looking systems. Honeywell introduced its RDR-4B Weather Radar, featuring 17 DSPs dedicated to windshear processing, or the equivalent processing power of a Cray-1 Supercomputer.
FLWS systems work on the same Doppler principle used in turbulence detection radars. A Doppler radar detects frequency shift which is proportional to the speed of the moving raindrops. In a non-turbulent rain shower, all of the raindrops are falling to the ground at about the same speed. In a turbulent rain shower, the updrafts and downdrafts create a difference in the speed of the raindrops. If this variance in the speed of the raindrops exceeds a certain threshold, a turbulence indication is displayed.
Looking at the windshear example below, the raindrops coming toward the aircraft are moving faster and the raindrops moving away from the aircraft appear slower. This fast to slow profile over a certain distance is called the windshear signature and is how the radar detects microbursts. Once the shear exceeds a pre-determined threshold an alert is generated based on location (azimuth and range).
Windshear Signature
F-Factor
It’s time to introduce some new terminology: F-Factor or Hazard factor. The easiest way to understand this is to think of an aircraft’s F-Factor as its energy state. The blue and purple bars on the graph below show several different types of aircraft in the takeoff and landing configuration.
The aircraft’s energy state is lower in the landing configuration with reduced power and gear and flaps down. The red bar shows the F-Factor of several measured microbursts (DFW, Charlotte, etc.). Simply put, if the microburst’s F-Factor exceeds that of the aircraft, it is a bad day.
What about lighter and more powerful corporate aircraft? Could they power through a large microburst encounter? No. The largest recorded microburst occurred right after Air Force 1 landed at Andrews AFB. The wind direction and speed changed 180 degrees and 109 knots! Few aircraft are likely to survive that scenario, nor could they out-climb the downdraft velocity at the core of a microburst, which can exceed 6,000 FPM.
What is different from the airlines is opportunity and location. The amount of regularly scheduled airline flights provides greater opportunity for windshear encounters. Flight history data shows that pilots are faced with such windshears on the average once every 2,200 flights and certain windshear hot spots exist around the world as shown below. The Boeing Business Jet and Airbus Corporate Jet, derived from commercial airliners, have windshear detection capability, and now, so do other bizjets and commuter aircraft in an effort to offer the same level of safety.
Wellington NZ: 1 every 100 landings |
Columbia SC: 1 every 150 landings |
Denver CO: 1 every 2,300 landings |
Dallas TX: 1 every 7,100 landings |
Windshear Encounter Odds
Reactive vs. Predictive Systems
The normal procedure for a reactive alert is to execute an escape maneuver. With an FLWS system the normal procedure is a go-around, but pilots should always consult the AFM and any OEM guidance as procedures can vary by aircraft.
It is the advanced warning time that saves the aircraft. This time is used to increase power and clean the aircraft up, increasing the aircraft’s energy state and climbing so that windshear is encountered at a higher, more survivable altitude where the windshear has not mushroomed out radially. Also, Doppler processing limits the windshear detection region to approximately +/-40 degrees ahead of the aircraft. It is not uncommon to have multiple microbursts in the same area, so a turn to avoid one microburst might place the aircraft in a banked turn and in position to encounter another possibly more severe microburst.
When windshear systems were being developed, NASA and the FAA evaluated several different maneuvers for windshear recovery. In pilot trials the difference between these maneuvers varied by only 20 feet. But when these maneuvers were delayed by just 5 seconds the average altitude loss increased by 300 feet! Again, it is the advanced warning time that saves the aircraft.
Dry Microbursts
As mentioned earlier, NASA studies showed that a minimum of 10 seconds warning significantly improved chances of recovery. The 10 seconds comes from the low reflectivity “dry microburst” case.
Dry microbursts are common in arid environments like Denver and Phoenix where the rain evaporates before reaching the ground (virga). A dry microburst is considered 0dBz reflectivity. To put it into perspective, the beginning of green on a radar display is 20 dBz or about 100 times more rainfall. To further put it into perspective, if there was a piece of paper on the ground, someone could count the raindrops as they fell during a 0 dBz rainfall. Fortunately, most microbursts are of the wet type and provide significantly greater reflectivity and warning times.
Part Two of this series willl talk about the types of alerts generated by the system.